Table of Contents
Introduction
The process of generating electricity using solar panels depends primarily on a crucial step. Electrons transition from the valence band (within the PN junction of the solar panel) to the conduction band (external circuit, such as a battery). Electrons that reside in the valence band, without external energy, are termed as such. To produce electricity, these electrons must move into the external circuit, known as the conduction band.
Electrons do not transition from the valence band to the conduction band on their own. A certain amount of energy (referred to as the band gap) must be provided to facilitate this transition.
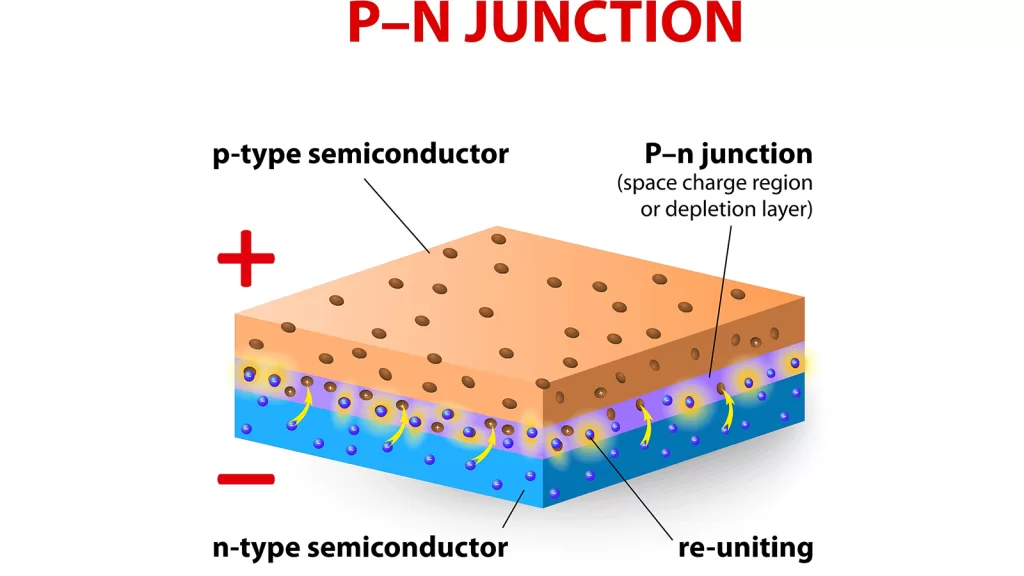
What Is A Band Gap?
The band gap is a critical concept in semiconductor materials, referring to the minimum energy required for electrons to jump to a higher energy level. This is akin to children needing enough force to hop to the next square in hopscotch. The size of the band gap determines the range of photon wavelengths a material can absorb, crucial for generating current in solar panels by efficiently absorbing photons across the solar spectrum. Varying band gap sizes enable materials to optimize photon absorption in high or low-energy light regions, adapting to diverse environmental and application needs.
For conductors, there is no gap between the conduction band and the valence band, so the conduction band is filled with electrons, making the material highly conductive. In contrast, insulators have a large gap between the valence band and the conduction band, preventing electrons in the valence band from jumping to the conduction band, which makes the material non-conductive. Semiconductors have a band gap that is between these two extremes, usually making them non-conductive. However, when energy is added (through light, heat, etc.), electrons in the valence band can move to the conduction band, allowing the material to conduct electricity.
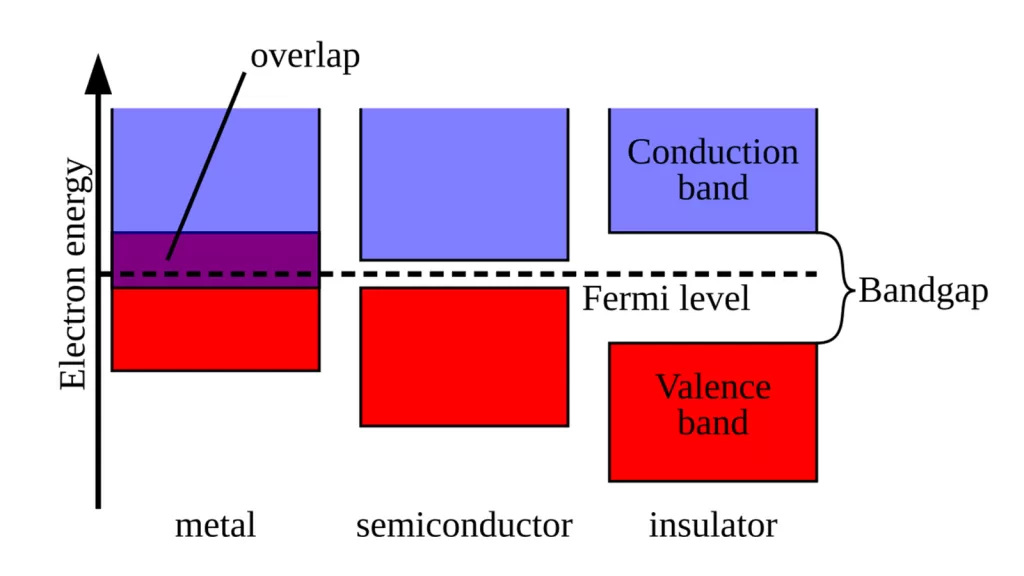
Why Is The Band Gap Important?
Solar cells work by absorbing energy from sunlight, which makes electrons jump to higher energy levels, creating an electric current. The band gap determines which energy particles (photons) in sunlight the solar cell can absorb. If the band gap is too large, many photons don’t have enough energy to make the electrons jump. If the band gap is too small, excess energy will be wasted. Therefore, the right band gap allows solar cells to convert sunlight into electricity more efficiently.
Why Is The Band Gap of 1.5 eV Considered Optimum?
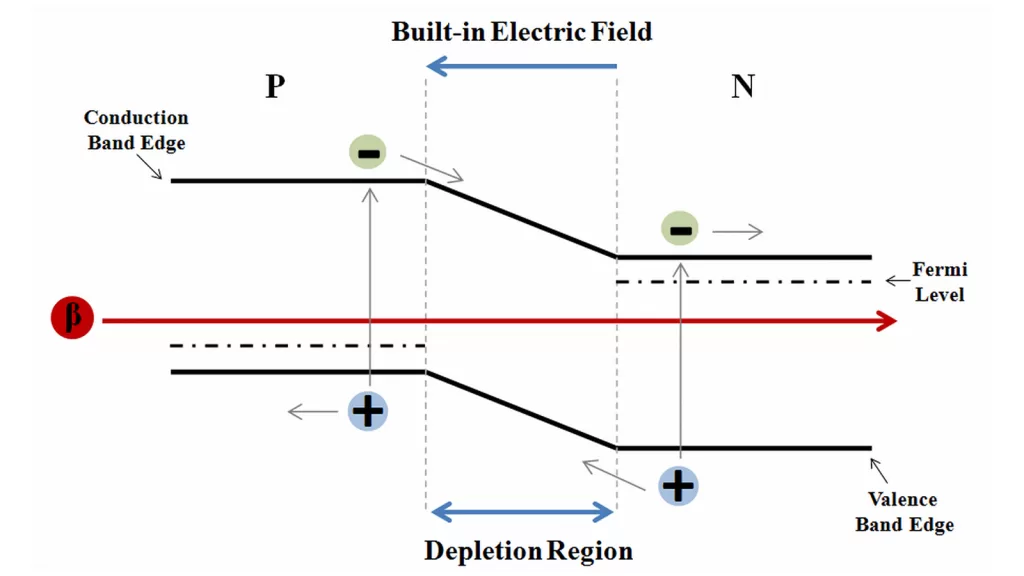
When photons excite electrons near the band gap of a semiconductor, three situations can occur:
1.When the energy of a photon is less than the semiconductor’s band gap energy, the electrons do not absorb the photon’s energy, and the photon passes through the semiconductor. This is called transparency loss.
2.If the photon’s energy is equal to the semiconductor’s band gap energy, the electrons absorb the photon’s energy and jump from the valence band maximum (VBM) to the conduction band minimum (CBM). The built-in electric field in the PN junction separates these electrons, fully converting the absorbed photon’s energy into electrical energy.
3.If the photon’s energy is greater than the semiconductor’s band gap energy, the electrons absorb the photon’s energy and jump to a position higher than the conduction band minimum (CBM). The excess energy is then released as heat through a process called relaxation, which is known as thermalisation loss. The electrons eventually fall to the conduction band minimum, and the built-in electric field separates them, converting part of the photon’s energy into electrical energy.
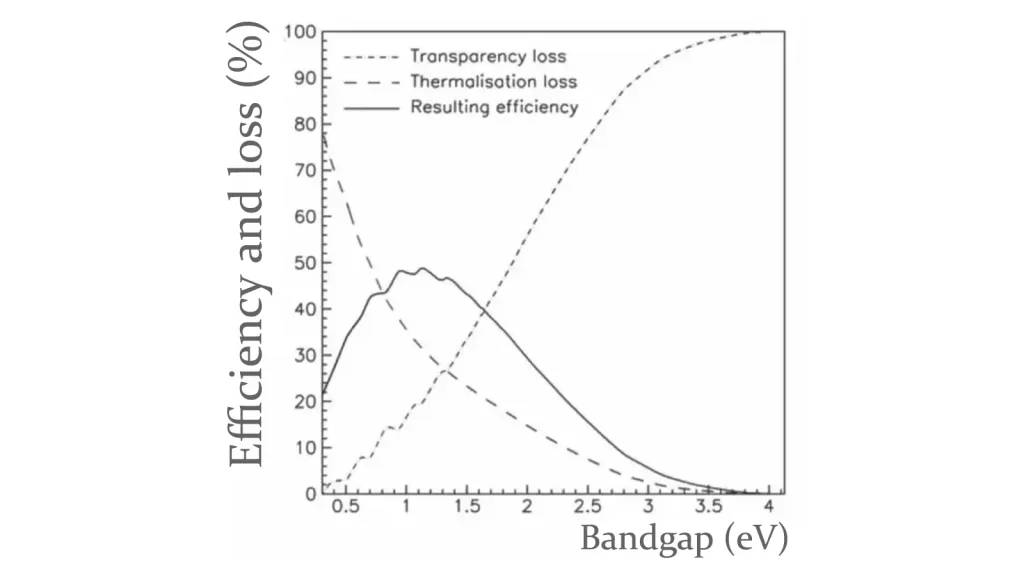
Based on the description above, we can draw the following conclusions:
1.A larger band gap means more low-energy photons can’t excite electrons from the valence band to the conduction band. Therefore, more photons are not absorbed, leading to greater transparency loss. Simply put, the larger the band gap, the greater the transparency loss. This is represented by the dashed line in the graph from the bottom left to the top right.
2.A smaller band gap results in more photons being absorbed. However, the excess energy from these photons is dissipated as heat through relaxation processes, increasing thermalization loss. In short, the smaller the band gap, the greater the thermalization loss. This is shown by the dashed line in the graph from the top left to the bottom right.
In the end, the actual efficiency of converting sunlight into electricity, η, can be described as:
η = 1 – Transparency loss – Thermalisation loss
The solid line on the graph shows this efficiency peaking in the middle and dropping at both ends. This is easy to understand: when the band gap is too large, almost no photons are absorbed, resulting in almost zero electricity conversion efficiency. Similarly, when the band gap is too small, most of the photon energy is lost as heat after being absorbed, also leading to nearly zero efficiency. The peak efficiency occurs somewhere in the middle, typically between 1.0 eV and 1.5 eV, as shown on the graph. It’s important to note that this graph may not represent conditions under the AM1.5 spectrum, where a band gap around 1.5 eV
Band Gap of Different Materials
1.Silicon
Silicon is one of the key materials for current mainstream solar cells. It has a band gap width of approximately 1.1 electron volts (eV), allowing it to effectively convert a wide range of sunlight wavelengths. The efficiency of silicon-based solar cells has been extensively validated, with laboratory tests showing monocrystalline silicon solar cells achieving up to 26.7% conversion efficiency, while commercial products typically reach around 20%. This material is well-suited for diverse global solar radiation conditions, offering excellent stability and a long lifespan. Data indicates that photovoltaic systems using silicon solar cells generally have a lifespan exceeding 25 years.
Since 2008, Maysun Solar has been dedicated to producing high-quality Silicon photovoltaic modules. Maysun Solar offers a variety of TOPCon, IBC, HJT solar panels, as well as balcony solar power stations. These solar panels boast excellent performance and stylish design, seamlessly integrating with any building. Maysun Solar has successfully established offices and warehouses in many European countries and has long-term partnerships with excellent installers! Please feel free to contact us for the latest module quotes or any photovoltaic inquiries. We are happy to assist you.
2.Perovskite
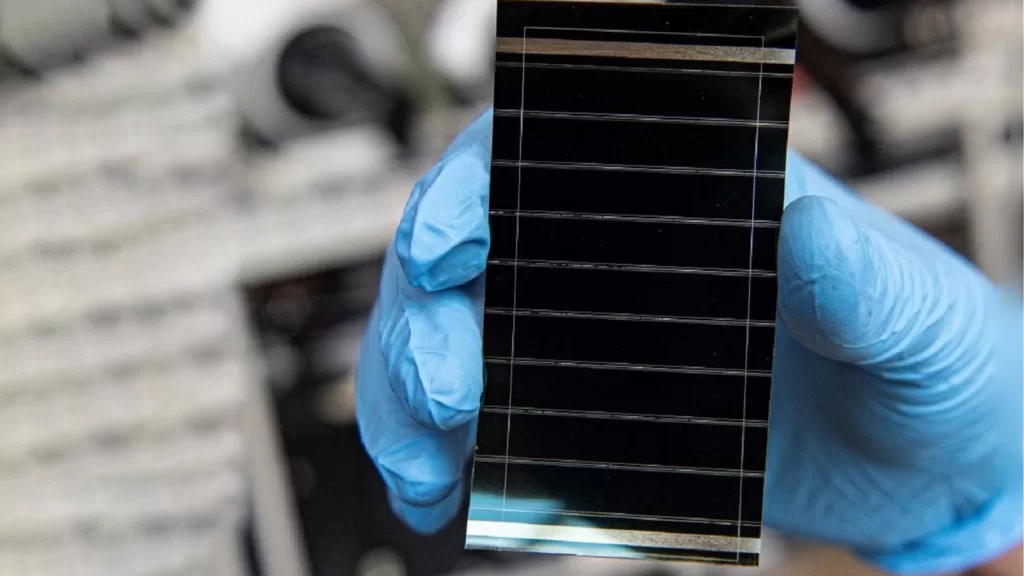
Perovskite materials can adjust their bandgap through chemical synthesis, offering potential to enhance efficiency and reduce manufacturing costs. Typically, the bandgap of perovskite solar cells ranges from 1.5 to 2.3 electron volts (eV), allowing them to efficiently absorb the visible light spectrum of sunlight. In recent years, these solar cells have seen a rapid increase in efficiency, rising from less than 4% in 2009 to over 25% today. They can be combined with silicon to form tandem solar cells, boosting overall efficiency and benefiting from low-temperature manufacturing processes that significantly cut production costs.
Researchers at the University of Cambridge focus on perovskite materials for flexible LEDs and next-generation solar cells. They have found that simplifying the chemical composition sequence can greatly enhance efficiency and reduce production costs. Currently, efforts are underway to address stability and environmental durability issues, aiming to facilitate their large-scale commercial application.
3.Other Materials
Scientists worldwide are researching advanced materials such as cadmium gallium selenide (CIGS), gallium nitride, germanium, and indium phosphide. These materials are designed to adjust the bandgap limits of multi-junction solar cells effectively, converting the entire spectrum of sunlight into electricity.
Cadmium gallium selenide (CIGS) and similar materials have a relatively narrow bandgap (approximately 1.0 to 1.7 electron volts, eV), allowing them to perform well under low light conditions. CIGS solar cells maintain high efficiency even on cloudy days and in weak light, making them particularly suitable for specific environmental conditions. For instance, in parts of Europe where sunlight intensity is lower throughout the year, CIGS solar panels demonstrate significant performance advantages. In laboratory conditions, CIGS solar cells have achieved conversion efficiencies up to 23.4%, while commercial products typically range from 15% to 18%. Additionally, CIGS materials are flexible and can be used to manufacture bendable solar cells, offering more choices for building-integrated photovoltaics and portable devices.
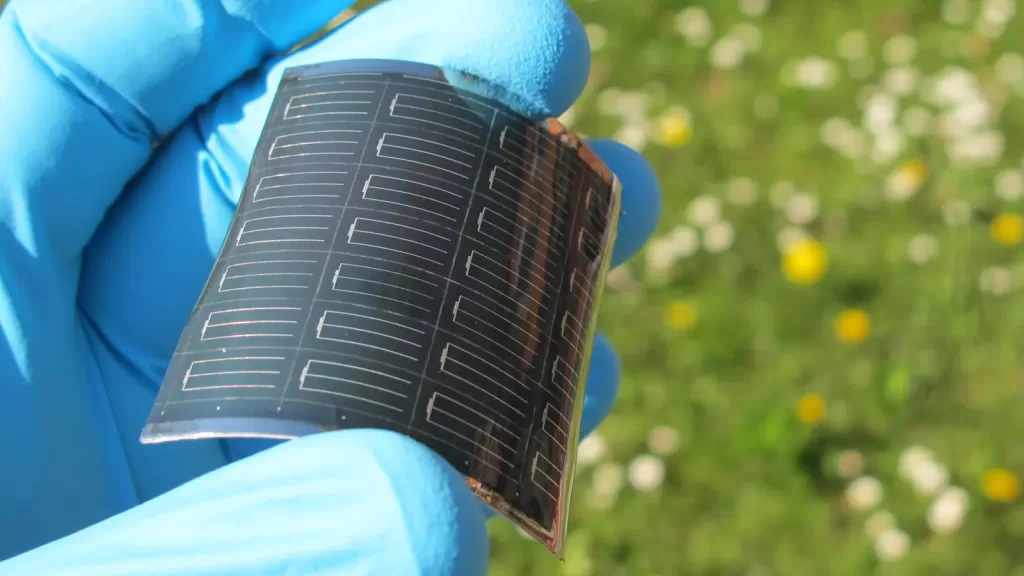
Band Gap Optimization and Practical Applications
Bandgap optimization is a key technology for enhancing solar cell performance. By precisely adjusting the bandgap of materials, significant improvements can be achieved in photovoltaic conversion efficiency and application versatility. In practical applications, the impact of bandgap optimization is evident in several aspects:
1.Enhancing Photovoltaic Conversion Efficiency:
Optimizing the bandgap of materials allows solar cells to more effectively absorb and convert photons across the solar spectrum. For example, employing multi-junction solar cell technology, which layers materials with different bandgaps, maximizes the absorption of different wavelengths of light, thereby significantly boosting overall efficiency. This technology has already achieved laboratory efficiencies exceeding 40% in space solar cells and high-efficiency terrestrial applications.
2.Adapting to Diverse Environmental Conditions:
Materials with different bandgaps are suitable for varying environmental conditions. Materials with wider bandgaps, like silicon, can operate stably under a wide range of solar radiation conditions, whereas narrower bandgap materials, such as CIGS, excel under low light conditions. Through bandgap optimization, solar cells can be designed to adapt to various climates and light conditions, enhancing their applicability globally.
3.Reducing Manufacturing Costs:
Bandgap optimization not only improves efficiency but also lowers production costs through material and process innovations. For instance, perovskite materials exhibit excellent bandgap tuning capabilities and cost-effective manufacturing processes, with rapid commercialization progress. Optimizing the bandgap can facilitate more efficient manufacturing processes, reducing cost per watt and enhancing market competitiveness.
4.Driving Development of New Photovoltaic Materials:
Bandgap optimization techniques are driving the development of new photovoltaic materials, such as organic-inorganic halide perovskites and quantum dot materials. These new materials, with precisely tuned bandgaps, demonstrate higher efficiency and broader application potential. In the future, these technologies are expected to enable innovative applications such as wearable photovoltaic devices and building-integrated photovoltaic systems.
Conclusion
In conclusion, bandgap optimization plays a crucial role in practical applications by not only enhancing the efficiency of solar cells but also improving their adaptability, reducing costs, and driving the development of new technologies. With continuous advancements in materials science and manufacturing processes, bandgap optimization will further propel the widespread adoption and advancement of solar energy technology, laying the foundation for the future of global renewable energy.
Reference:
Solar Cells: A Guide to Theory and Measurement. (n.d.). Ossila. https://www.ossila.com/pages/solar-cells-theory
Why are solar cells so inefficient? (n.d.-c). http://m.myjizhi.com/1000000000665023
Niclas. (2024, February 22). Energy Band Gap of Solar cells. Sinovoltaics (Hong Kong Office). https://sinovoltaics.com/learning-center/solar-cells/energy-band-gap-of-solar-cells/
Explained: Bandgap. (2010, July 23). MIT News | Massachusetts Institute of Technology. https://news.mit.edu/2010/explained-bandgap-0723
Read more:
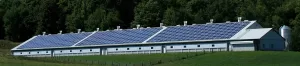
Empowering Factories with Solar Energy A Strategic Tool for Controlling Production Electricity Costs
Commercial and industrial solar is becoming a key solution for factories to reduce electricity costs and hedge against price fluctuations. This article systematically analyzes its deployment models, cost advantages, and sustainable value pathways.
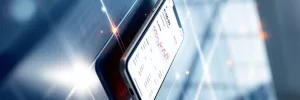
How Businesses Can Offset Carbon Taxes with Solar Power
This article analyzes the latest carbon tax policies and photovoltaic deduction strategies, helping European businesses legally reduce taxes, increase profits through solar investment, and achieve a win-win situation for both economy and environment.
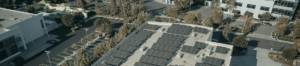
Forecast and Response: Seizing the Next Decade’s Growth Dividend in Europe’s Commercial and Industrial Photovoltaics Market
Maysun Solar analyzes the growth trends of commercial and industrial photovoltaics in Europe over the next ten years, from policies and ESG to technological innovation, helping companies seize the initiative in the energy transition.
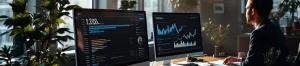
How to Calculate Solar System ROI and Optimize Long-Term Returns?
Solar power is becoming a key solution for businesses to reduce costs and improve efficiency. Accurately calculating ROI and optimizing long-term returns are essential to maximizing investment value.
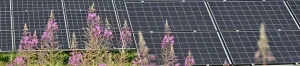
Will Agrivoltaics Affect Crop Growth?
Agrivoltaics combines solar energy and agriculture to reduce up to 700 tons of CO₂ per MW, improve water use, and boost crop growth for sustainable farming.
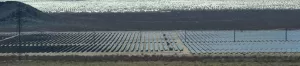
6.5 Billion Loss Hits Photovoltaics: Reshaping or Elimination?
In 2025, the photovoltaic market may see a turnaround as some companies take early action. A €6.5 billion loss is driving businesses to explore new growth areas like energy storage and hydrogen. Which giants will break through? Industry transformation is accelerating!