Microcracks within solar panels are minuscule fractures or fissures that can emerge within the photovoltaic cells or the protective layers of the solar panel structure. These fractures, although often microscopic and undetectable to the naked eye, play a crucial role in influencing the overall performance and lifespan of solar panels. Microcracks may affect the performance of the solar panel, resulting in a loss of power, a much shorter service life, or even termination of the energy production of the entire solar panel. This article explains the causes of microcracks in solar panels, how they are detected, their effects, and what types of solar panels are less likely to produce them.
Table of Contents
Why Are There Microcracks on My Solar Panels?
The causes of microcracks can be diverse and multifaceted:
Defects in manufacturing:
Improper practices during the manufacturing process can significantly contribute to the occurrence of microcracks in solar panels. Inadequate handling, suboptimal material selection, or lapses in quality control create an environment where vulnerabilities are introduced. These vulnerabilities, stemming from the manufacturing stage, become potential catalysts for the initiation and propagation of microcracks within the solar panel structure. Therefore, it underscores the critical need for meticulous attention to detail, proper training of personnel, and stringent adherence to quality control protocols throughout the entirety of the manufacturing process to minimize the risk of microcrack formation.
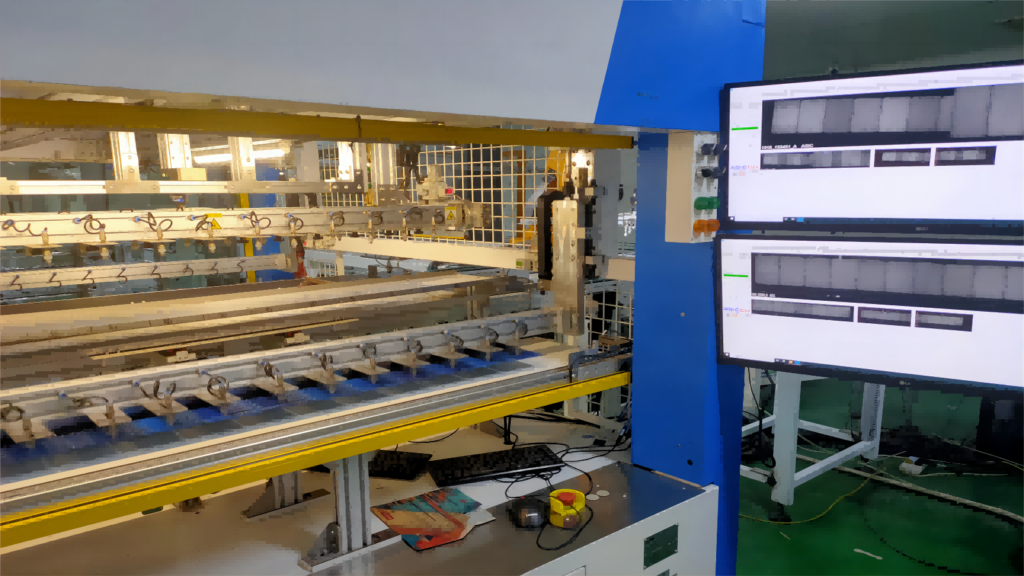
Mechanical stress:
Mechanical stress is a significant factor contributing to the formation of microcracks in solar panels, and it can be categorized into various phases, including transportation, installation, and external environmental factors.
During transportation, mechanical stress can significantly impact solar panels, particularly when it comes to packaging, storage, and handling practices in the supply chain. Inappropriate packaging or storage procedures may subject solar panels to excessive pressure, potentially resulting in the formation of microcracks. Improper transportation methods, including rough handling or improper stacking, can subject solar panels to vibrations and impacts, resulting in mechanical stress and an increased risk of the formation of microcracks.
Therefore, addressing issues related to packaging, storage, and handling is crucial to minimizing the potential for microcrack formation during the transportation phase.
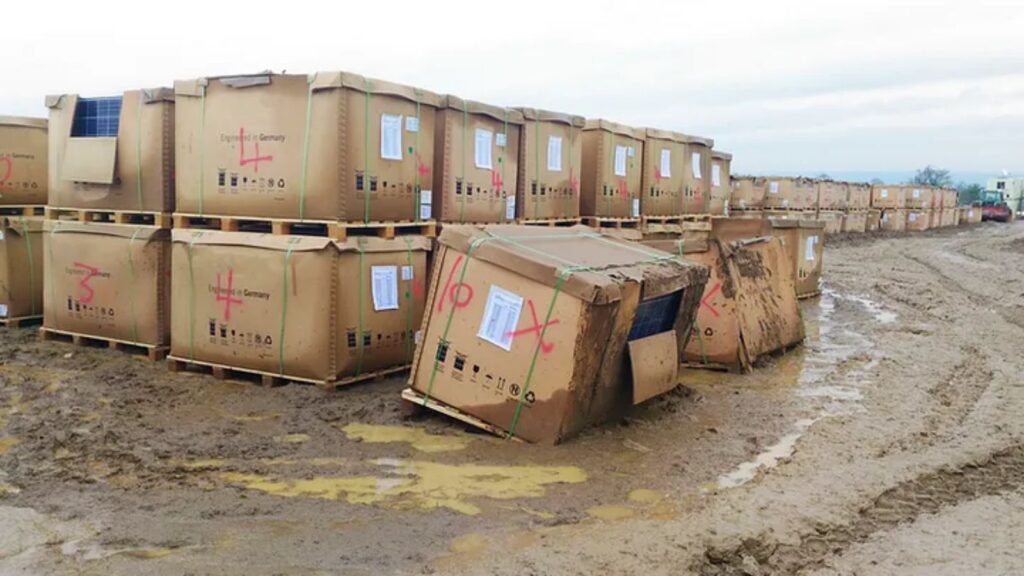
Installation-induced mechanical stress is also a critical concern for solar panels. Practices like improper stacking, resting equipment on panels, accidental bumps, and nonplanar surface installation can create localized pressure points, leading to microcrack formation. Dragging panels during transport introduces friction-induced stress, while improper carrying techniques, like using only one person or sitting on the module edge, can cause uneven forces. These practices pose a risk to the efficiency and longevity of solar panels, emphasizing the need for careful handling and installation procedures to mitigate mechanical stress and ensure optimal performance.
As shown in the figure below, it was concluded from testing that incorrectly resting the shoulders and head on the panel during stair climbing resulted in a 1% power drop. The proper way to carry the panel is to balance the weight in your hands against the panel frame.
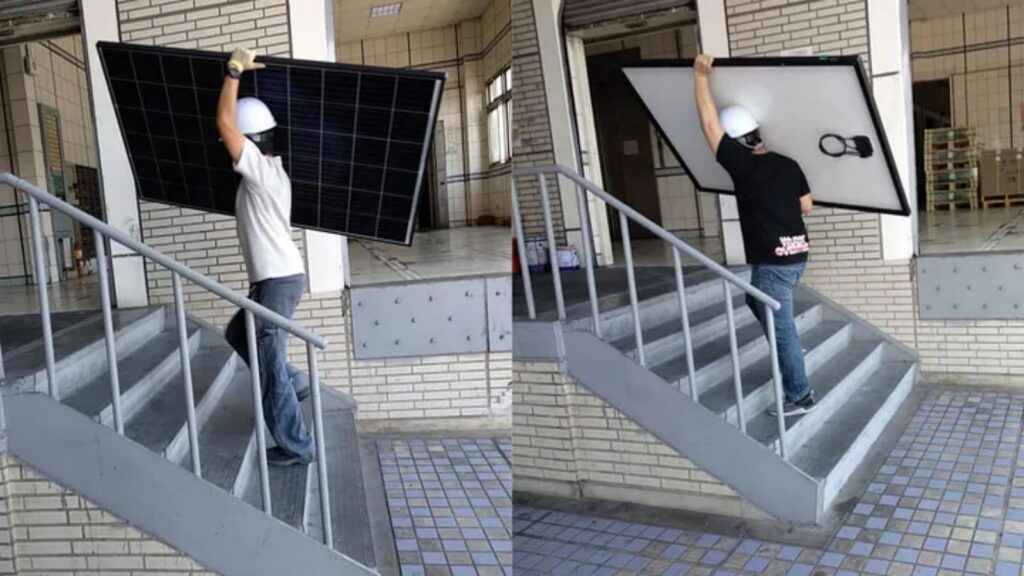
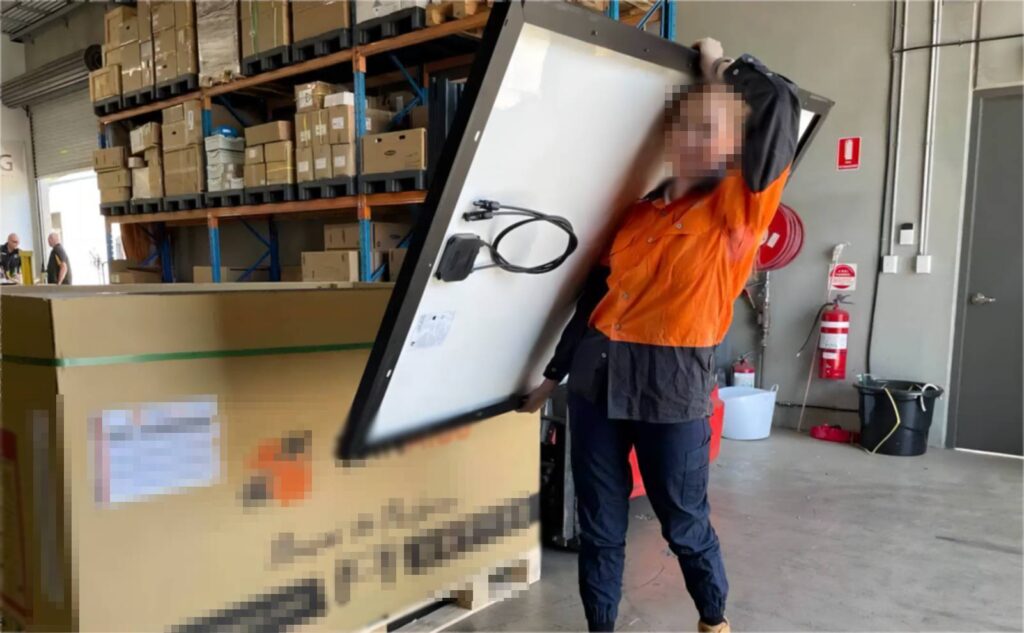
During the long-term operation of solar panels, external environmental factors, including weather-related stress such as wind, hail, and extreme temperature variations, play a significant role in imposing mechanical stress on solar panels. These environmental stressors, when persistent over time, can contribute to the cumulative development of microcracks. Therefore, a comprehensive approach to minimizing the risk of microcrack formation in solar panels involves understanding and mitigating mechanical stress at various stages, from transportation, installation and operation. By addressing both internal handling practices and external environmental factors, the overall reliability and performance of solar panels can be enhanced.
Thermal cycling:
Thermal cycling, which results from the daily and seasonal fluctuations in temperature, emerges as a pivotal factor contributing to the development of microcracks in solar panels. The constant expansion and contraction of materials within the solar panels, influenced by varying sunlight and weather conditions, establish conditions favorable for the initiation and propagation of microcracks over extended periods of exposure. The repetitive stress induced by thermal cycling underscores the importance of considering temperature variations in the design, manufacturing, and installation processes of solar panels to enhance their durability and longevity.
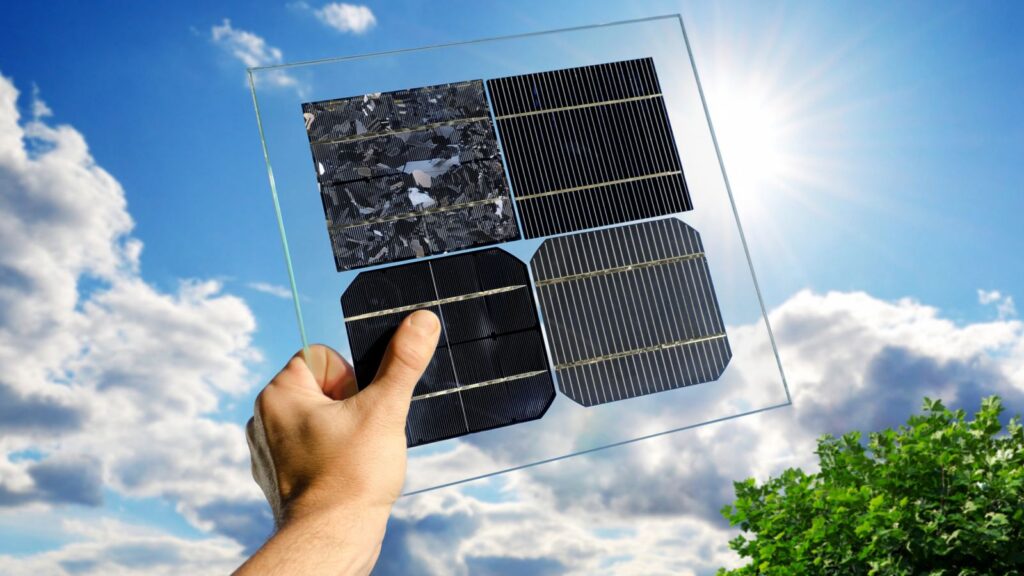
What Damage Can Be Caused by Microcracks?
Increased Resistance and Thermal Damage: Microcracks can cause a rise in electrical resistance, elevating temperatures and creating hotspots. This escalation in thermal damage further contributes to the overall deterioration of the affected solar cell. In certain instances, microcracks can propagate, leading to the isolation of a significant area within an individual cell from the circuit. This isolation may result in reverse bias, inducing elevated temperatures in the connected section of the cell. As temperatures rise, there is a risk of causing permanent damage, not only diminishing energy production but, in extreme cases, potentially leading to catastrophic failure, such as glass breakage or, in unfortunate instances, fire due to intense heat generated by a cell in reverse bias.
Reduced Power Output: The increased electrical resistance leads to a decrease in power generation, causing a decline in the overall efficiency of the solar panel.
Crack Propagation and Module Failure: If microcracks extend in length, they may evolve into larger cracks, potentially halting or terminating the energy production of the entire module. This outcome necessitates replacement and significantly impacts the overall lifespan of the solar panel.
To mitigate these detrimental effects, early detection through regular inspections is essential. Various methods, as mentioned earlier, can be employed to identify and address microcracks promptly, ensuring the sustained performance and longevity of solar panels.
How to Inspect for Microcracks During the Installation and Operation of Solar Panels?
Detecting microcracks in solar panels is a pivotal process essential for ensuring optimal performance and prolonged longevity. Various inspection methods cater to distinct scenarios during both installation and operation phases:
EL (Electroluminescence):
Electroluminescence (EL) operates on the principle of generating light when an electric current is applied to a material. In the realm of solar cells, EL is applied to visualize and identify defects not easily visible through alternative methods. EL testing proves particularly effective in detecting micro-cracks, broken cells, and other internal defects within solar cells. This method offers direct insight into the structural integrity of cells, uncovering hidden issues that might escape detection by other testing methods.
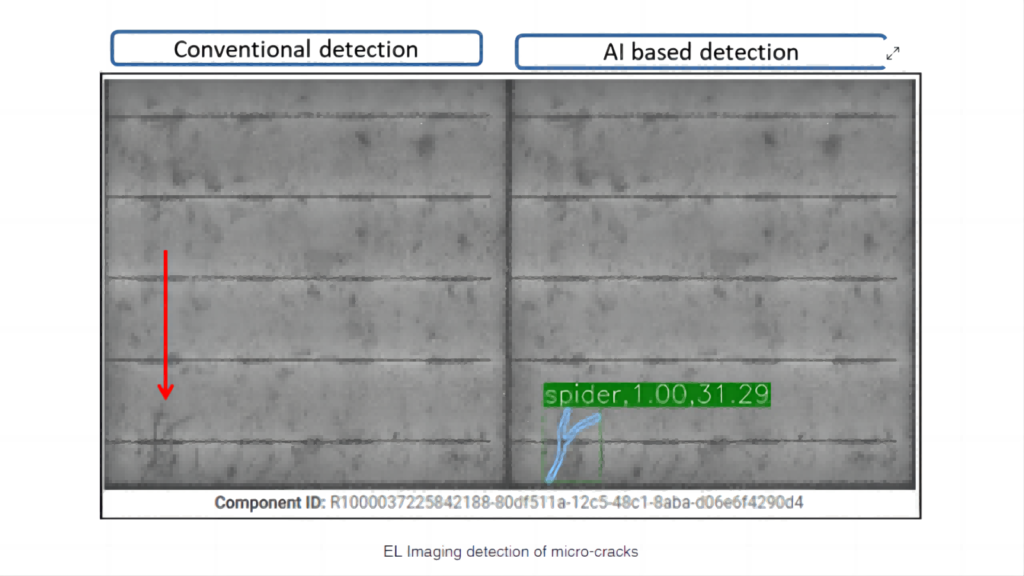
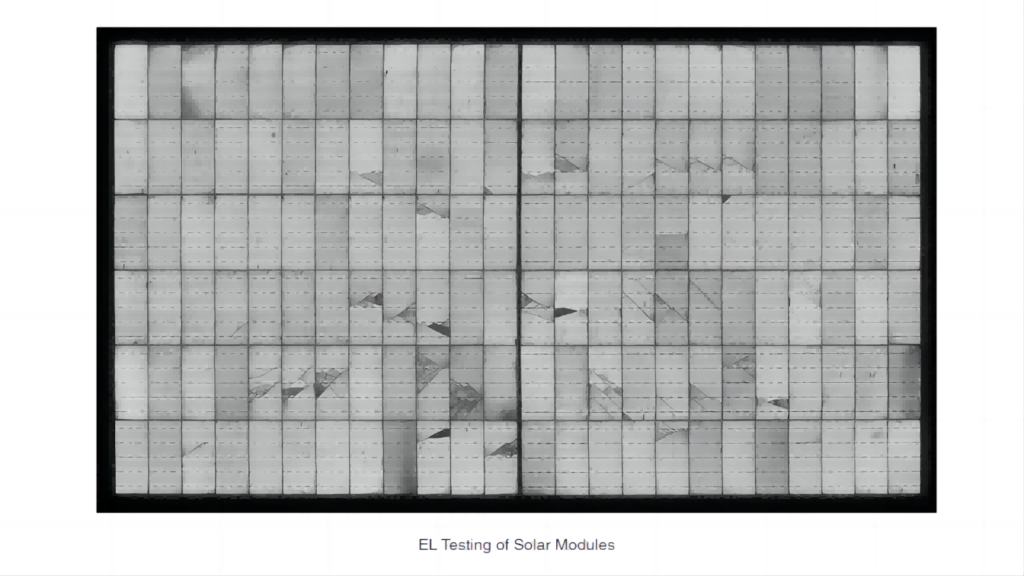
ELCD (Electroluminescence Crack Detection):
ELCD utilizes electroluminescence to efficiently detect microcracks in solar panels. Applying an electric current activates electroluminescent materials, producing visible light that is altered by micro-cracks, making them visually detectable. This non-destructive method is crucial for quality control during manufacturing and preventive maintenance in the field. Unlike traditional Electroluminescence (EL), ELCD is specifically designed for microcrack detection, offering enhanced precision in pinpointing and visualizing these minute structural defects. The specificity of ELCD makes it a valuable tool, ensuring the reliability and efficiency of solar panel installations by addressing issues that might go unnoticed by other testing methods.
In addition to these two ways, there are several other methods:
Photoluminescence Imaging:
Best utilized during installation and operation, photoluminescence imaging identifies microcracks affecting photovoltaic conversion efficiency. This technique seamlessly integrates into regular maintenance routines to uphold ongoing efficiency.
Infrared Imaging:
Infrared imaging (IR) operates by capturing the thermal radiation emitted by an object, allowing for the identification of temperature variations indicative of defects or irregularities. Specifically in solar cells, IR is widely utilized for thermal inspections to detect overheating, identify malfunctioning components, and spot irregularities in both solar cells and photovoltaic modules. This technique is particularly effective in identifying issues associated with electrical connections, such as loose or damaged wiring.
Visual Inspection and Microscopic Examination:
Most applicable during installation and routine maintenance, visual inspection and microscopic examination involve direct observation and detailed structural scrutiny. This hands-on approach effectively identifies visible cracks on solar panel surfaces.
In practice, professionals may opt to employ these inspection methods individually or in combination, depending on the specific requirements and circumstances of the solar panel system. It is imperative to engage trained experts, especially when utilizing advanced technical equipment, to ensure precise detection and analysis of microcracks. Regular monitoring and a comprehensive approach contribute significantly to the overall reliability and longevity of the solar energy system.
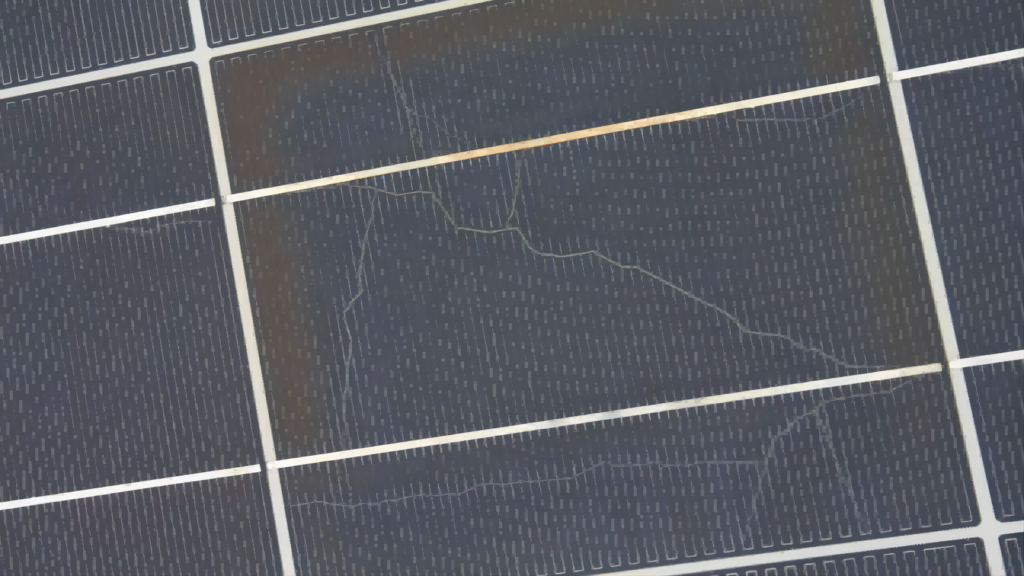
Choose the Right Solar Panels To Reduce Microcracks
In the field of solar panels, managing microcracks is crucial for ensuring system reliability and performance. While microcracks pose a common challenge, innovative technologies, such as IBC technology and HJT technology, have significantly reduced the impact of microcracks on performance, the benefits of their resistance to microcracks are specified in the following points:
HJT Solar Panels:
Type Monocrystalline Silicon Substrate:
HJT solar cells employ an N-type monocrystalline silicon substrate known for its high purity and uniformity. Compared to polycrystalline silicon, the monocrystalline structure is more compact and consistent, helping to reduce microcracks caused by stress concentration.
Undoped Amorphous Silicon Layer (i-a-Si:H)
A layer of undoped amorphous silicon is deposited on the monocrystalline silicon substrate, acting as a “buffer layer.” This layer provides additional elasticity and protection when the cell undergoes physical stress, such as bending or pressure during transportation and installation. This helps to alleviate stress on the substrate, thereby reducing the formation of microcracks.
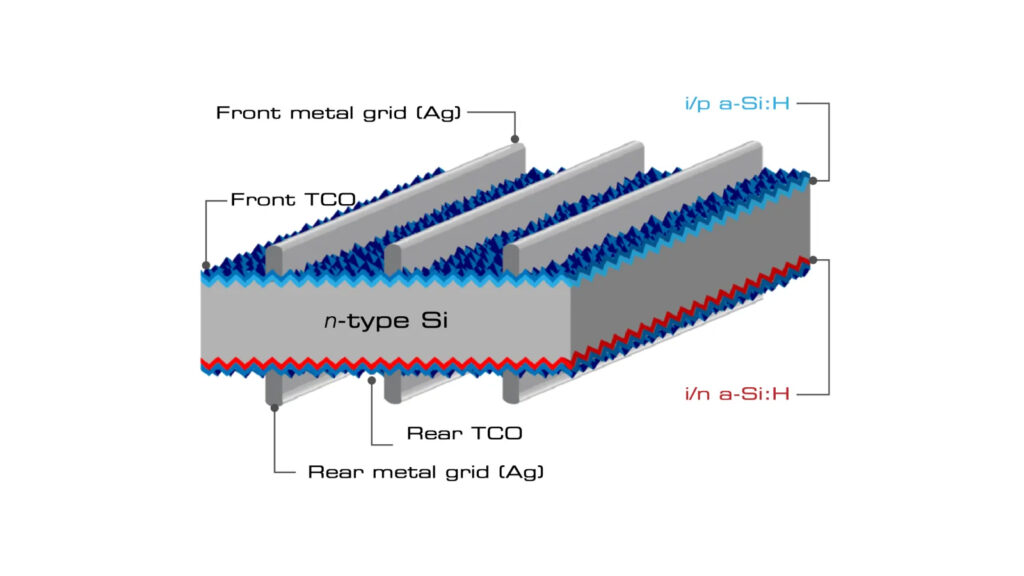
Complete Half-Cell Processing Method:
HJT solar panels utilize a unique complete half-cell processing technique that entirely avoids cutting damage to the solar cells. This innovative approach significantly minimizes the impact of microcracks on solar panels, proactively addressing potential harm during manufacturing and handling processes.
Lower Manufacturing Temperature:
Compared to traditional crystalline silicon solar cells, HJT cells are produced at lower temperatures. This reduced processing temperature means the materials undergo less thermal stress during production, aiding in the reduction of microcrack formation.
Optimized Overall Structure:
The overall structure of HJT cells is optimized during design and manufacturing to enhance their resistance to physical stress. For instance, the edges and corners of the cells are designed to be smoother, reducing stress concentration points.
Ultra-Multi Busbar Design:
HJT panels feature a design with narrower busbars, reducing the amount of silver paste used and consequently decreasing shading effects while shortening current transmission distances. This design not only improves the electrical performance of the panels but also minimizes the current loss caused by microcracks, damaged busbars, and fractures, further enhancing the panel’s reliability and lifespan.
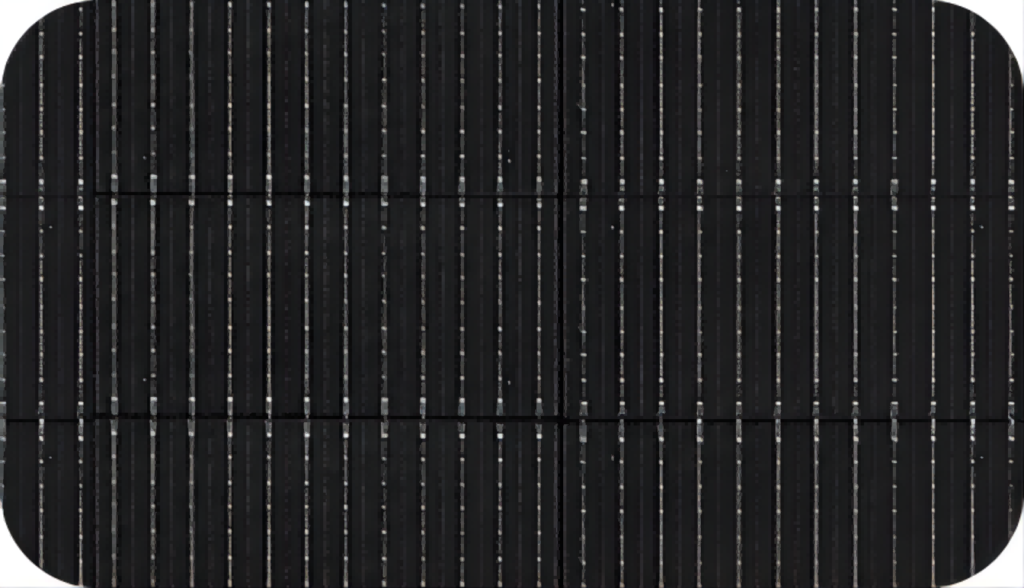
Enhanced Mechanical Strength:
Through the various design and manufacturing optimizations mentioned above, the overall mechanical strength of HJT solar cells is enhanced. This increased strength helps maintain the integrity of the cells during transportation and installation, reducing the risk of microcracks.
Maysun Solar harnesses the unparalleled advantages of heterojunction technology (HJT), resulting in our HJT solar panels making a significant advancement in solar power generation. These panels not only achieve a quantum leap in efficiency but also exhibit a notable improvement in resistance to microcracks.
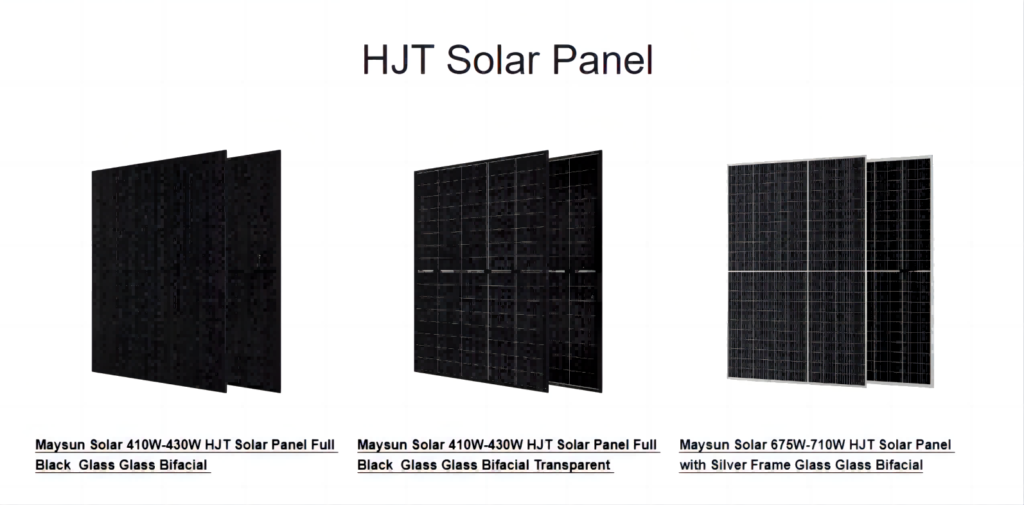
IBC solar panels have a significant advantage in weather resistant. The innovative All Back Contact design incorporated in IBC solar panels prevents tensile damage and detachment attributed to the thermal expansion and contraction of the front welding strip under severe temperature stress. This design feature guarantees the consistent and stable power generation of the solar panels, mitigating the adverse effects of thermal variations on panel integrity.
Maysun Solar’s latest generation of interdigitated Back Contact (IBC) solar panels seamlessly integrate microcrack resistance, high efficiency, aesthetic appeal, and an extended operational lifespan. Choosing Maysun Solar’s IBC solar panels represents a judicious decision for individuals aiming to maximize both efficiency and performance. The image below shows the IBC 430W Full Black solar panels installed on a roof by a German customer from Maysun Solar. For additional information, kindly click the button below.
Maysun Solar has been specialising in producing high quality photovoltaic modules since 2008. Choose from our wide variety of full black, black frame, silver, and glass-glass solar panels that utilise half-cut, MBB, IBC, HJT and Shingled technologies. These panels offer superior performance and stylish designs that seamlessly blend in with any building. Maysun Solar successfully established offices, warehouses, and long-term relationships with excellent installers in numerous countries! Please contact us for the latest module quotations or any PV-related inquiries. We are excited to assist you.
Reference:
Chang, T. (2023, July 31). How microcracks damage solar panels – WINAICO Australia. WINAICO Australia. https://www.winaico.com.au/blog/what-cause-microcracks
RenewSys India. (2023, February 3). Micro cracks in solar modules: Causes, detection and prevention. renewsysworld. https://www.renewsysworld.com/post/micro-cracks-in-solar-modules-causes-detection-and-prevention
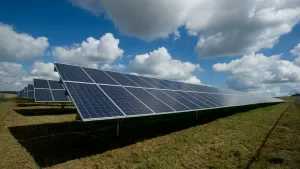
Dual Benefits: How Agrivoltaics Helps Farmers Increase Income
Agrivoltaics generates income for farmers through solar energy, while also enhancing crop yields and land use efficiency. European policies support this model with subsidies, net metering, and flexible electricity sales, making agrivoltaics a sustainable income source and a new driver of agricultural development.
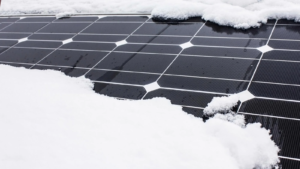
How to Prevent Snow from Reducing Solar Panel Efficiency?
Learn how to prevent snow and ice buildup on solar panels to maintain efficiency and maximize energy production during winter. Discover practical methods and safety tips to keep your solar system working well in snowy conditions.
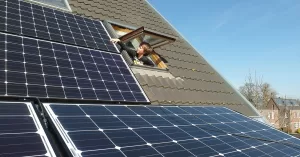
A Comprehensive Analysis of Whether Photovoltaic Systems Emit Radiation
This article provides a thorough analysis of electromagnetic radiation in photovoltaic systems, addressing health concerns. It compares the radiation levels of PV systems with household appliances, highlighting the negligible impact of PV radiation on human health. It also offers optimization tips for safe usage.

Best Practices for Installing Bifacial Solar Panels
Discover the benefits of bifacial solar panels and learn how to optimize their installation for maximum efficiency. This article covers different panel types and essential best practices, including ideal height, spacing, site selection, etc.

The Bifaciality of Solar Panels: A Comprehensive Guide from Principles to Applications
Learn about bifacial solar panels and the concept of bifaciality, explore the different types of bifacial modules available in the market and their applications, compare them with monofacial modules, analyze the factors influencing the power generation gain of bifacial modules, and understand their widespread applications across various fields.
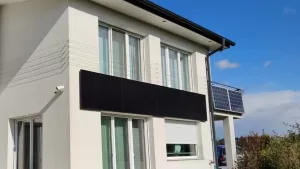
Can solar panels be installed vertically?
Discover the pros and cons of vertical solar panels, compare them with rooftop installations, and learn about their investment returns and payback periods, along with answers to common installation questions.