Introduction:
In the field of renewable energy, solar technology has shown to be a game-changer, providing important solutions for lowering carbon emissions and boosting energy sustainability. Solar panels, sometimes referred to as solar modules, are an essential component of solar systems since they control how much energy is generated. In order to achieve the best system performance, choosing the appropriate solar panel is an essential step.
This article explores each element affecting solar panels’ energy output. You will be better able to design and plan your solar system, meeting your energy needs while maximizing the effectiveness of sustainable energy generation, if you completely comprehend these essential components.
Table of Contents
How does solar module’s operating current and operating temperature affect electricity generation?
When the operating current of a solar module is higher, it typically leads to an increase in the module’s operating temperature. This is because the current’s magnitude is related to the generation of heat within the module due to internal resistance, and higher currents result in more heat losses.
Heat losses cause an increase in the temperature of the solar module. At higher temperatures, the flow of electrons slows down, reducing voltage, and consequently, the efficiency of the solar module decreases.
To study the relationship between the electricity generation performance of different modules and their operating temperatures, JinkoSolar, in cooperation with TUV Nord, conducted an outdoor empirical project at the Yinchuan National Photovoltaic Experimental Base in February 2021. The operating temperatures of the ultra-high current modules (18A) were, on average, approximately 1.8°C higher than those of the 182 modules (13.5A), with maximum temperature differences of around 5°C. This is primarily because the excessive operating current of the modules leads to a significant increase in heat losses on the surface of the solar cells and soldering ribbons, contributing to the rise in the module’s operating temperature. As widely known, the output power of PV modules decreases with an increase in temperature. For instance, in the case of PERC modules, when the module temperature exceeds the rated operating temperature, the power output decreases by approximately 0.35% for every degree Celsius increase in temperature. Considering a combination of factors, the empirical results show that the 182 modules achieve a single-watt electricity generation rate approximately 1.8% higher than that of the ultra-high current modules. Maysun’s Twisun black frame modules offer the advantage of low current (9A) and high power, performing better in high-temperature conditions because the low current helps reduce operating temperatures, decrease heat losses, and improve module efficiency.
The following images illustrate the comparison of operating temperatures between the ultra-high current modules (18A) and the 182 modules (13.5A)
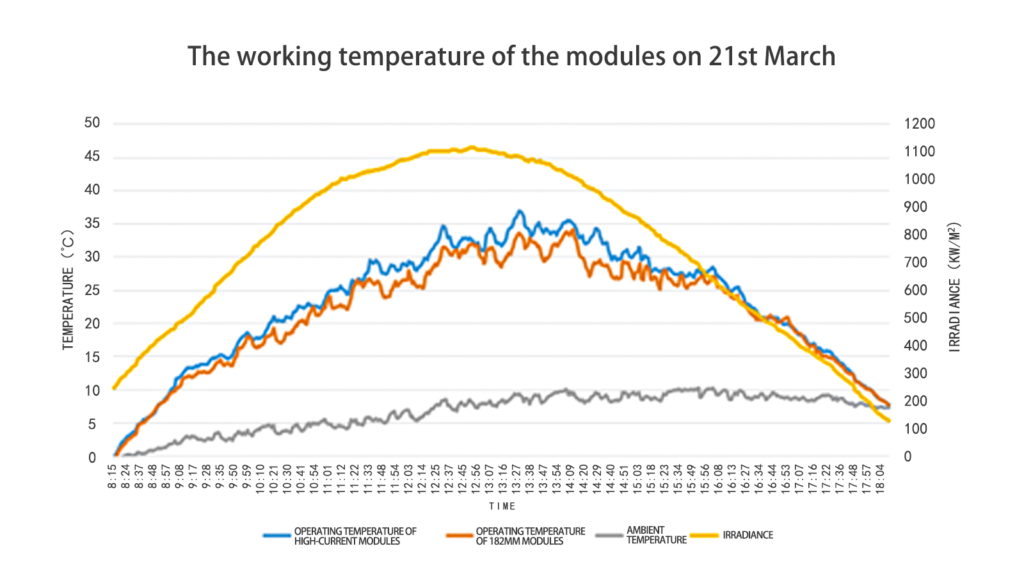
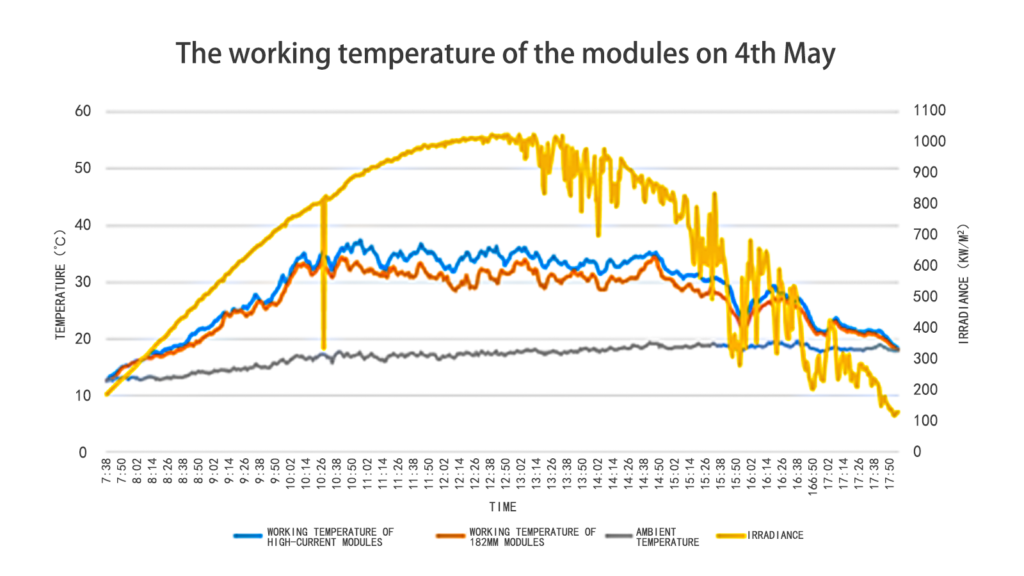
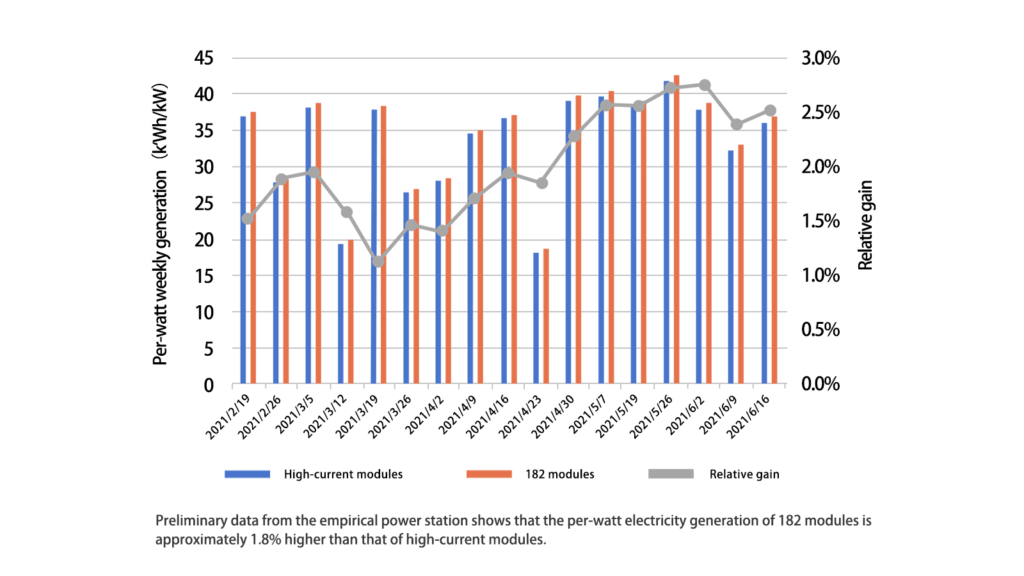
The preliminary data from the empirical station shows that, on March 21st and May 4th, the operating temperatures of the ultra-high current modules (18A) and the 182 modules (13.5A) were measured. The operating temperatures of the ultra-high current modules were noticeably higher than those of the 182 modules. An increase in temperature leads to a reduction in electricity generation. The 182 modules achieve a single-watt electricity generation rate approximately 1.8% higher than the ultra-high current modules.
Suggestion:
Large current modules can lead to increased thermal losses, causing them to heat up more and, in turn, resulting in a more substantial drop in their output power. It’s imperative to enhance the thermal loss control of solar panels. Implementing cooling measures, such as mounting heat dissipation plates beneath the modules or elevating the height of the solar panels from the ground for improved ventilation, can be beneficial.
Furthermore, when choosing inverters and solar panels, ensuring that the panel’s Maximum Power Point Current (often abbreviated as MPP current) does not exceed the inverter’s Maximum Power Point Tracking (or MPPT) maximum input current is crucial. This is because the inverter’s MPPT circuit needs to effectively track the solar panel’s MPP to maximize energy conversion efficiency. For instance, if an inverter’s MPPT is rated at 12.5A and a panel’s MPP current is at 13.5A, then the module would not be compatible with that inverter.
Why the Temperature Coefficient of Solar Modules Matters?
The temperature coefficient of solar modules is a vital performance parameter, indicating the performance variation of solar panels under different temperatures. The rated power of solar modules is determined under Standard Test Conditions (STC). If, during operation, the actual running temperature exceeds the rated working temperature, the output power will decrease. This is because the photovoltaic conversion efficiency of the module decreases as the temperature rises. For instance, if the power temperature coefficient is -0.34%/°C, for every 1°C rise above the rated working temperature, the module’s output power will reduce by 0.34%.
Moreover, temperature fluctuations also impact the long-term stability and lifespan of solar modules. Elevated temperatures can lead to material fatigue within the modules, reducing their longevity. Typically, modules with a lower temperature coefficient are more likely to have a longer lifespan. In extreme cases, overheating of solar modules could pose safety risks, even leading to fires.
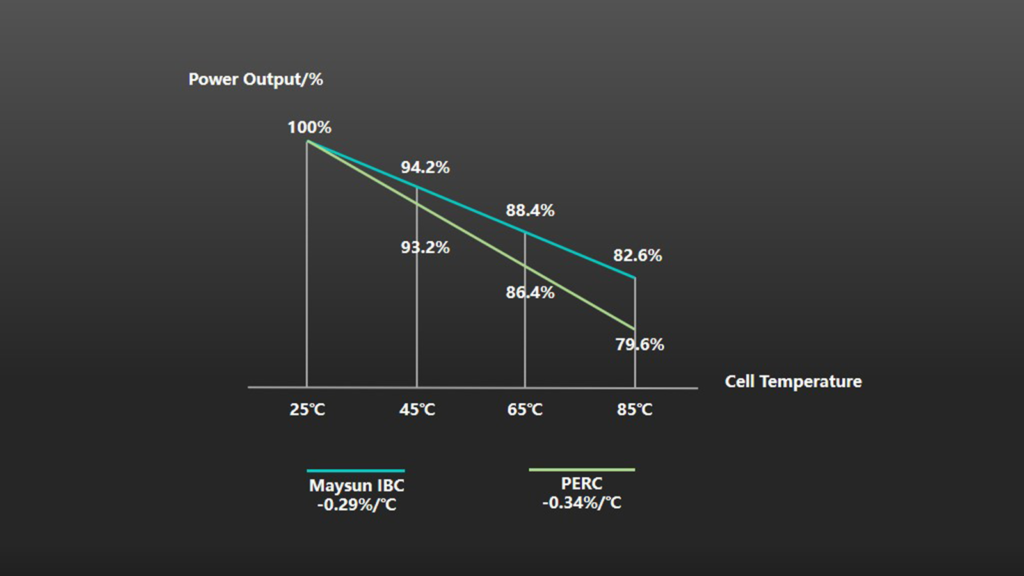
According to chart data, the temperature coefficient for Maysun’s IBC modules stands at -0.29%/℃. This means that for every 1℃ increase in the IBC module’s working temperature, there’s a power reduction of 0.29%. On the other hand, PERC modules have a temperature coefficient of -0.34%/℃. This indicates that for each 1℃ rise in the PERC module’s working temperature, the power drops by 0.34%. In high-temperature environments, where the module’s operating temperature can reach 85℃, the PERC module’s power has significantly dropped to 79.6%, whereas the IBC module can still maintain an output of 82.6%.
Suggestion:
Therefore, when in hotter regions or when considering safety under high-temperature conditions, opting for modules with a lower temperature coefficient is a prudent choice. IBC (Interdigitated Back Contact) solar panels, with their lower temperature coefficient( -0.29%/℃), exhibit a distinct advantage in high-temperature areas.
Spectral Response:An Essential Performance Metric
Solar cells harness the photoelectric effect to convert sunlight directly into electricity. Their spectral response delineates the range of light spectrum they can efficiently cater to. Currently, most of the solar cells available in the market are silicon-based, primarily responsive to the visible spectrum and a portion of the infrared radiation. In contrast, their responsiveness to ultraviolet and a significant part of the infrared spectrum is relatively weak.
The provided picture demonstrates a typical solar radiation spectrum alongside the spectral response of a silicon solar cell. It’s essential to understand that this spectral response, or spectral sensitivity, defines the radiation range at which the cell operates most effectively. This profoundly influences its efficiency under varied radiation conditions. These cells predominantly respond to the visible spectrum and the near-infrared.
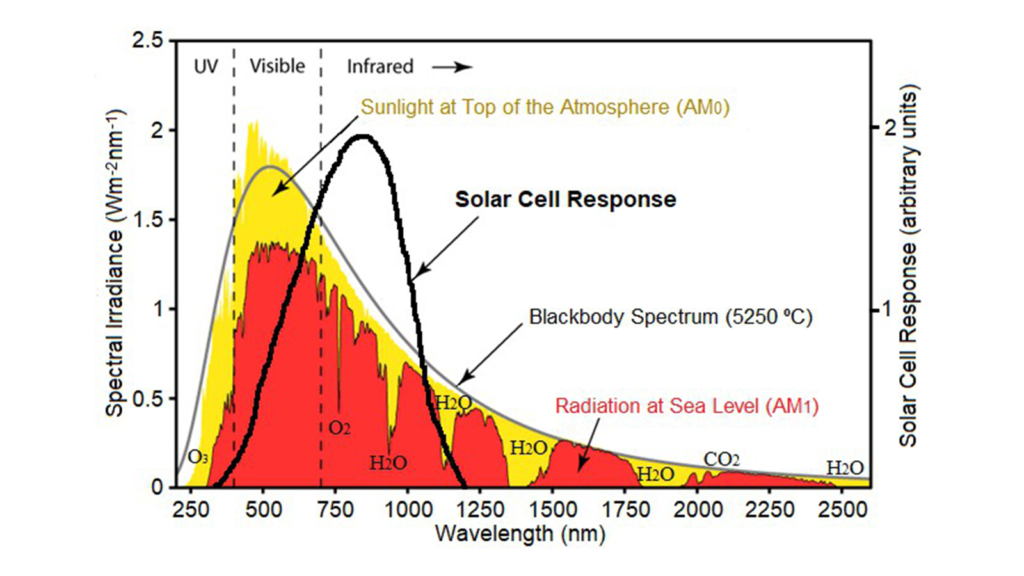
Diving into the Spectral Response Characteristics of a Typical Silicon-Based Solar Cell:
Visible Light Response: Silicon-based solar cells exhibit robust responsiveness to visible light, mainly concentrated within the 400-700nm wavelength range. Within this spectrum, the energy from light can stimulate valence electrons in silicon atoms, promoting them to the conduction band, resulting in the formation of electron-hole pairs, thereby producing current.
Short-Wavelength Infrared Response: These cells show a certain responsiveness to the shorter wavelengths of infrared light, chiefly concentrated between 800-1100nm. Light within this spectrum can promote electrons within silicon atoms to the conduction band, enhancing the current output.
Ultraviolet Light Response: Silicon-based solar cells’ responsiveness to ultraviolet light is relatively subdued, primarily occurring within the 200-400nm wavelength spectrum. The energy from this part of the spectrum is too meager to stimulate valence electrons in silicon atoms to the conduction band, resulting in minimal current generation.
Long-Wavelength Infrared Response: The responsiveness to the long-wavelength part of the infrared spectrum is also limited, primarily between 1100-1200nm. The energy in this spectrum is too low to generate sufficient current.
Given the same solar module, the energy output can vary substantially across regions with significant differences in their light spectrum. Monocrystalline silicon solar cells exhibit a superior quantum efficiency compared to polycrystalline silicon cells, especially in the 310-550 nm spectrum. In this range, the quantum efficiency of monocrystalline silicon cells can even surpass that of polycrystalline cells by over 20%, resulting in higher power generation.
Suggestion:
Before embarking on solar power plant construction, it’s prudent to select modules with a broader spectral response based on the predominant irradiance bands in the locale. Compared to other technology modules, IBC modules boast a vast spectral response, capable of capturing solar radiation ranging from ultraviolet to visible light and up to the near-infrared spectrum, approximately between 300nm to 1200nm. This extensive range ensures that IBC modules perform exceptionally well under various illumination conditions, including low-light and diffused light scenarios.
Low-Light Performance and Its Impact on Energy Output
The term “weak light effect” in the context of solar panels refers to their performance and energy output under low illumination. This is commonly observed during the early mornings, late evenings, overcast days, or when a portion of the panels is shaded. The weak light effect has a significant bearing on the solar system’s overall performance and energy generation capabilities.
Under conditions of dim light, the diminished intensity means that the electrons within the solar panels move at a reduced pace, leading to a drop in current generation and a notable decline in the panels’ energy output. Concurrently, the solar modules take a longer duration to achieve the operational voltage required by inverters, thereby curtailing the effective power generation hours of a photovoltaic system within a day.
Suggestion:
To counteract this, it’s prudent to opt for solar modules that excel under low-light conditions, such as the IBC (Interdigitated Back Contact) modules or the HJT (Heterojunction) modules. IBC cells, with their unique back-contact structure, are adept at capturing diffused light from the sides and rear, offering a distinct advantage when light conditions fluctuate or are inherently low, making them particularly suited for high-latitude regions. On the other hand, HJT modules, owing to their heterojunction design, boost charge separation and collection efficiency. This makes them ideal for generating efficient power output under cloudy skies or during early mornings and late evenings.
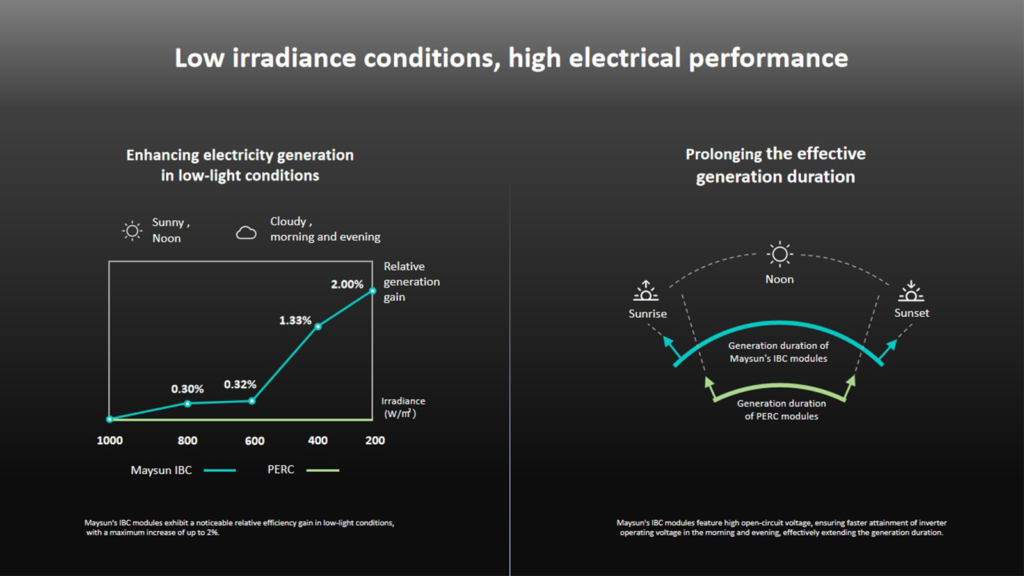
According to data from the TUV SUD certification testing center, Maysun’s IBC solar modules exhibit minimal compound central loss. In weak light conditions, when juxtaposed with PERC products, there’s a clear efficiency gain. At an irradiance level of 200W/m², IBC modules demonstrate a relative power gain of 2.01%. Additionally, due to the high open-circuit voltage characteristics of IBC modules, they reach the inverter’s operational voltage more quickly during early mornings and evenings, effectively prolonging power generation duration.
How Does Module’ Degradation Occur?
Degradation reactions of a module include PID (Potential Induced Degradation), LID (Light-Induced Degradation), LeTID (Light and elevated Temperature Induced Degradation), UVID (UV Induced Degradation), aging, and the hotspot effect. These degradation reactions are performance degradation processes that solar panels may undergo under specific conditions, affecting power output and the long-term performance of the system.
(1)PID:
Potential Induced Degradation (PID) refers to the performance degradation of solar panels under specific voltage differences. PID occurs due to the challenge of maintaining a long-term seal on photovoltaic modules during use, particularly under alternating high temperatures and humidity. This can lead to significant charge accumulation on the surface of the cell, impacting passivation and resulting in efficiency decline, with power generation potentially dropping by more than half.
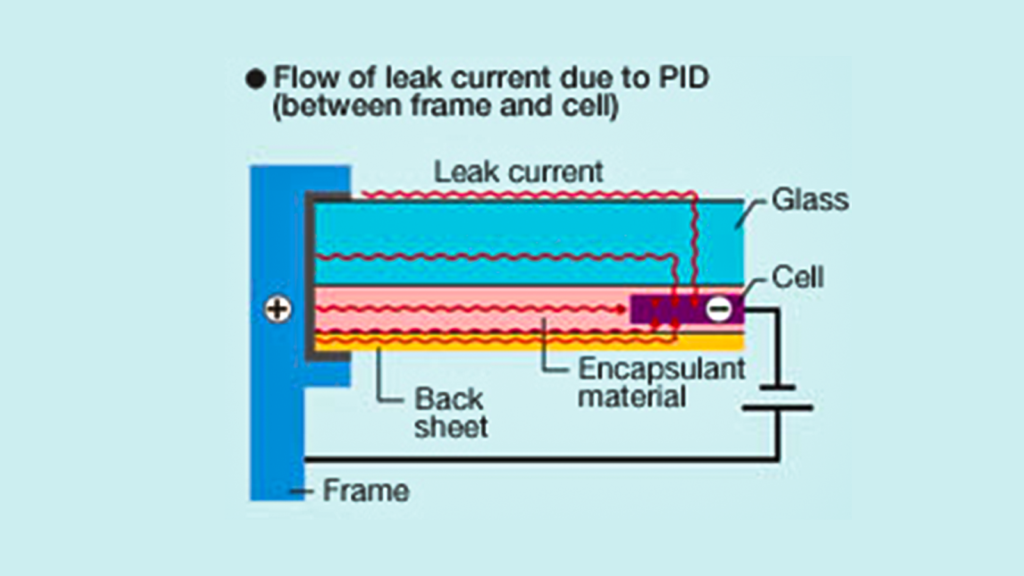
Ways to reduce the PID effect:
From long-term experiments, Maysun’s product experts have summarized methods to mitigate PID. They primarily involve:
Grounding the negative terminal of serial components or applying a positive voltage between the module and the ground during the evening.
Enhancing the lifespan and quality of EVA film and optimizing the encapsulation process.
Modifying the cell’s emitter and SiN anti-reflection layer.
Maysun’s HJT module developed possesses excellent anti-PID performance. Its TCO (Transparent Conductive Oxide) thin film layer has conductive properties, preventing charge polarization on the surface, structurally avoiding PID degradation.
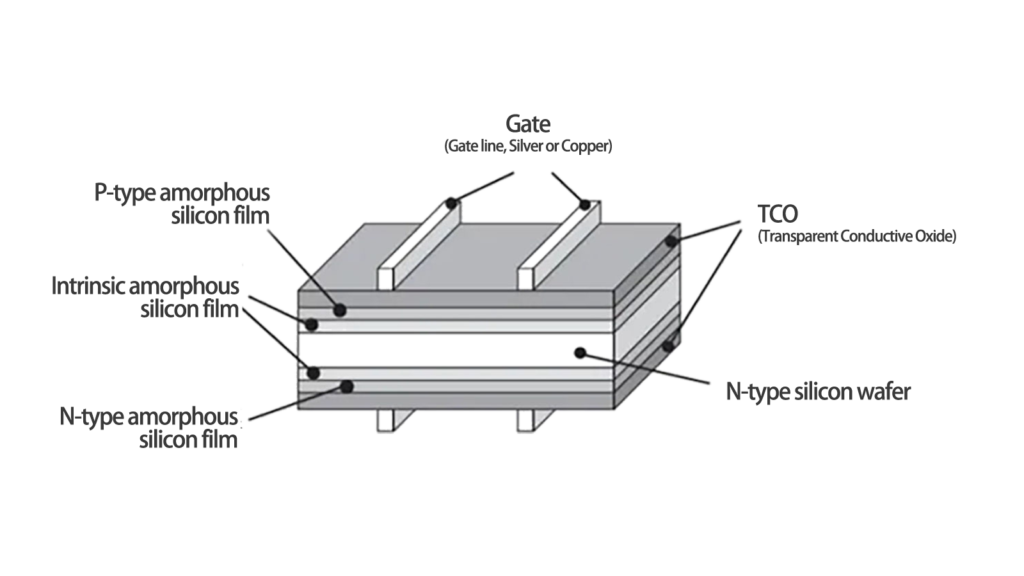
(2)LID:
LID (Light-Induced Degradation) is a reliability parameter for photovoltaic modules. It generally comprises three main types: Boron-Oxygen compound light degradation (BO-LID), light and elevated temperature-induced degradation (LeTID), and ultraviolet-induced surface passivation degradation (UVID).
BO-LID (Boron-Oxygen compound light degradation): Typically, when we speak of LID, we are referring to BO-LID, considered the primary factor for light degradation in crystalline silicon cells. As soon as photovoltaic modules are exposed to sunlight, LID begins, and in a short period (days or weeks), it can reach saturation. Resolving BO-LID can be achieved by modifying the dopants (like introducing Gallium) or improving passivation techniques.
LeTID (Light and Elevated Temperature Induced Degradation): LeTID is a thermally induced performance loss, mainly associated with materials and defects in solar cells. Under high temperature and radiation, defects in the cell can increase, leading to charge recombination and increased resistance, consequently reducing the cell’s performance. LeTID effects are usually observable during the actual operation of the module, not under lab conditions. To mitigate LeTID effects, manufacturers often improve material choices, fabrication processes, conduct thermal stability tests, and evaluate cell performance under high temperatures to ensure consistent module performance.
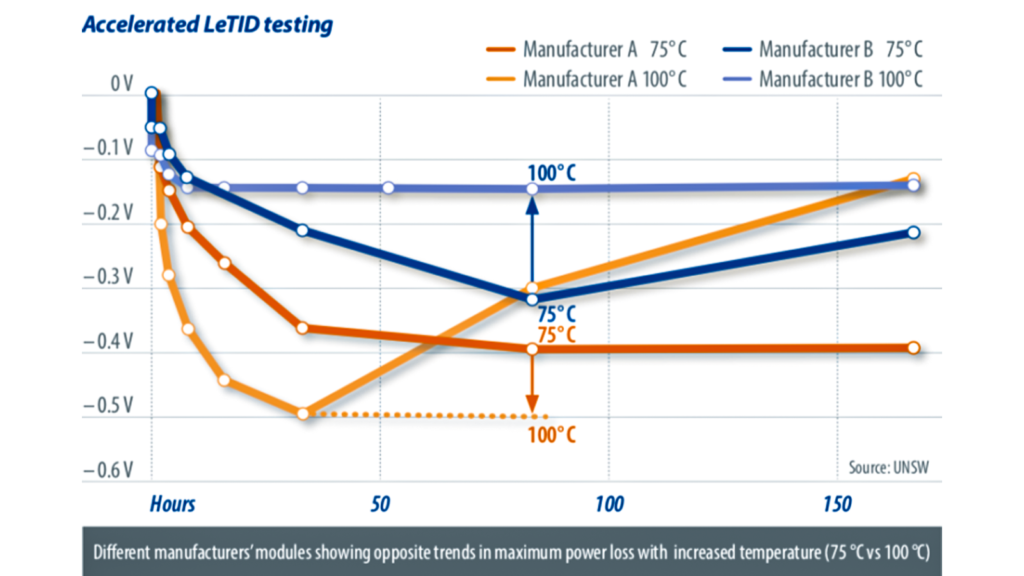
UVID (Ultraviolet Induced Degradation): UVID pertains to the potential performance decline in solar modules upon prolonged exposure to ultraviolet radiation. This degradation primarily links with the materials used in solar cells, especially photoelectric conversion materials. Continuous UV exposure can lead to chemical reactions or disintegration within the cell materials, causing performance degradation, often manifesting as reduced efficiency and power output. To combat UVID effects, manufacturers typically utilize materials with high UV stability, enhance the module’s encapsulation materials for better protection, and conduct UV exposure tests to gauge module robustness.
Currently, Maysun’s HJT (Heterojunction Technology) modules have managed to achieve a no LID effect. Due to the HJT cell substrate, which is typically N-type monocrystalline silicon and doped with phosphorus, there’s an absence of boron-oxygen and boron-metal complexes found in P-type silicon. Thus, HJT cells are immune to LID effects.
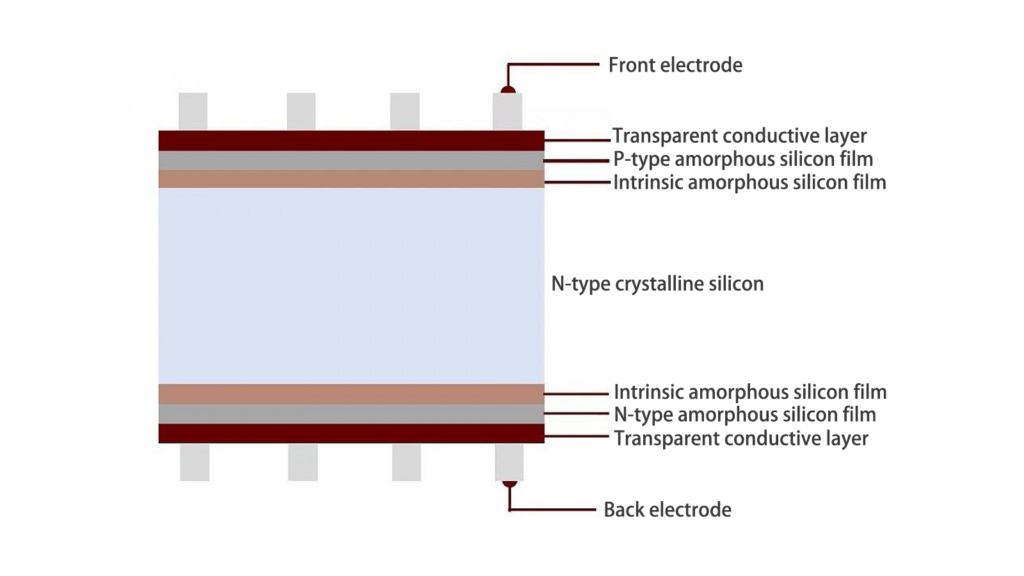
(3)Solar Module Aging
Solar modules, pivotal in capturing solar energy, aren’t immune to time and environmental wear. As they age, their efficiency may wane, leading to diminished energy outputs. Here, we break down the primary factors affecting module longevity:
Encapsulant Yellowing: Prolonged UV exposure can cause the encapsulant within modules to yellow, impacting both appearance and light-absorbing capability. This can reduce the module’s overall conversion efficiency.
Backsheet Wear: Over time, especially under high temperatures and humidity, the backsheet’s moisture resistance might degrade, increasing the risk of encapsulant hydrolysis and cell corrosion.
Cell Performance Decline: Continuous operation in challenging conditions can reduce solar cell efficiency and power outputs due to material property changes.
Manufacturers are aware of these challenges. For example, Maysun’s IBC solar modules come with a 25-year power and product warranty. They promise only a 1.5% efficiency drop in the first year and a mere 0.4% annual linear decline thereafter, ensuring users gain consistent benefits throughout the module’s lifespan.
(4)Hot Spot Effect
The hot spot effect refers to a potentially adverse situation in solar panels where certain cells or parts of the module tend to heat up more than others. This can compromise the performance and safety of the entire module.
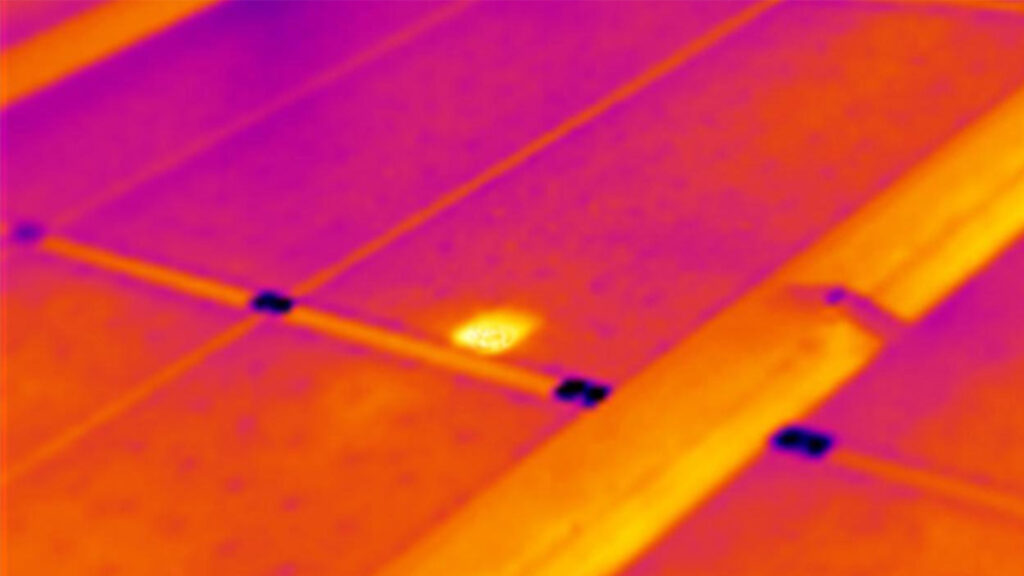
When does the hot spot effect occur?
Shading or Obstruction:
If a portion of a solar panel is shaded or obstructed, those particular cells won’t produce current, but the adjacent cells will continue to function. This forces the shaded cells to act as a load, absorbing heat from the neighboring functioning cells, which can cause them to become excessively hot.
Cell Inconsistencies:
Sometimes, there can be minor discrepancies or imperfections between solar cells. This can cause certain cells to heat up faster than their counterparts, leading to hot spots in those specific areas.
Implications of the Hot Spot Effect:
Cell Damage:
Hot spots can degrade or damage the overheated cells, potentially reducing their lifespan and performance.
Safety Concerns:
Elevated temperatures due to hot spots can pose fire risks or other safety hazards.
To mitigate the hot spot effect, Maysun Solar has integrated MOS bypass switches in their Venusun series panels, replacing traditional bypass diodes. These switches offer a quicker response to varying light conditions, adapting swiftly and minimizing the impact of shading on module performance.
Impact of Installation Methods and Solar System Accessories on Power Generation:
Factors concerning installation methods and solar system accessories include the tilt angle of the solar panels, combination losses of the panels, cables, transformer losses, controllers, inverter efficiency, and more.
- Tilt angle of solar panels:
The tilt angle of solar panels has a direct correlation with the amount of electricity generated. It refers to the angle at which panels are mounted on their brackets, affecting how they receive sunlight. The optimal tilt angle depends on the latitude of the location and the specific design of the system. General guidelines are as follows:
- Latitude 0°–25°: Tilt angle equals latitude.
- Latitude 26°–40°: Tilt angle equals latitude plus 5°–10°.
- Latitude 41°–55°: Tilt angle equals latitude plus 10°–15°.
(2) Combination losses of solar panels:
In a solar PV array, panels can be connected in series or parallel. When connected in series, losses can occur due to current mismatches between panels. When connected in parallel, losses result from voltage mismatches between panels. Combination losses can reach over 8%. Moreover, inconsistencies in the degradation characteristics of panels can result in voltage and current mismatches over the long term, reducing the overall power output of the PV system.
Suggestion:
Therefore, when installing a PV system, it’s advisable to use solar panels of the same brand and model. This ensures that the working current, voltage, and degradation characteristics of the panels are as consistent as possible. Isolation diodes can also be installed in the solar panels to prevent reverse current flow. This can mitigate any negative impact on the entire array due to shaded or damaged panels caused by sub-optimal solar system accessories.
(3) Cable and Transformer Losses:
One of the key factors ensuring the efficient operation of a solar energy system is managing line losses. Line losses refer to the percentage of electrical energy lost during transmission due to wire resistance, connectors, and other factors. Keeping line losses below 5% is a reasonable goal to ensure that system performance isn’t significantly compromised.
Suggestion:
To reduce line losses, it’s advisable to select wires and cables with good conductivity. Copper wires are typically preferred due to their excellent conductive properties. Additionally, the cross-sectional diameter of the wire is an important factor. Wires with a larger diameter have lower resistance, which can help in reducing line losses. It’s also essential to ensure that connectors and terminals are securely installed and tightly connected to reduce resistance and current losses. Furthermore, minimizing cable lengths and having an efficient layout can also help in reducing line losses.
Transformer losses refer to the energy lost during the transmission and distribution of electrical energy due to the internal resistance of transformers, magnetic losses, and other factors. These losses can influence the final delivery and distribution of generated electricity.
Suggestion:
To minimize the impact of transformer losses on power generation, opting for highly efficient transformer technologies that reduce internal losses is recommended. Regular maintenance and inspections of transformers ensure their optimum performance.
(4) Controller and Inverter Efficiency:
Inverters, containing inductors, transformers, and power devices like IGBTs and MOSFETs, experience losses during operation. Typically, string inverters have an efficiency of 97-98%, while centralized inverters have an efficiency of 98%. Losses within inverters arise from inductors, transformers, power devices, and other components. Equipment malfunctions leading to downtimes in inverter devices can also affect power generation. Transformer efficiency is usually very high, over 99%, resulting in almost negligible energy losses. The voltage drop in the charging and discharging circuit of the controller should not exceed 5% of the system voltage.
Suggestion:
It’s crucial to conduct regular maintenance of the solar system accessories to ensure the smooth operation of controllers and inverters, thereby reducing the occurrence of malfunctions.
How Does the External Environment Impact Solar Panel Efficiency?
Environmental elements like solar irradiance, shading, dust, extreme temperatures, hail, and precipitation can all influence the performance and lifespan of solar panels.
Solar Irradiance
Solar irradiance is the primary energy source for solar systems. It varies based on geographical location, season, and weather conditions. Geographical positioning determines the angle and duration of sunlight exposure, while seasons and weather conditions influence atmospheric factors, such as cloud cover and humidity, subsequently impacting the efficiency of solar panels. Under optimal sunlight conditions, solar panels can produce more energy. The irradiance intensity typically follows this pattern: winter, summer, spring, and then autumn.
Suggestion:
Plan and design your solar system based on the specific geographical location, climate, and energy needs to ensure optimal solar efficiency across varying seasons and temperatures. Furthermore, opt for solar panels with high efficiency and superior performance under low-light conditions, such as IBC (Interdigitated Back Contact) or HJT (Heterojunction) panels. These panels generate more energy under similar lighting conditions.
Shading Losses
Shading from trees or structures can decrease solar panel efficiency. Such shading can account for up to a 5% loss in energy generation. Factors like dust accumulation, snow deposition, or debris like leaves and bird droppings, if not cleaned in time, can not only decrease the system’s energy output but also lead to localized heating spots. Persistent localized heating, known as hot spots, can potentially damage the glass surface.
Suggestion:
When installing solar systems, choose locations with minimal shading from trees or buildings. For large-scale ground installations, consider using tracking systems to follow the sun’s movement, minimizing shading losses. Regular cleaning and maintenance are essential to ensure the solar system operates at peak efficiency.
Extreme Weather Conditions
High temperatures can elevate the working temperature of solar panels, reducing their efficiency and accelerating their aging process. Rain or snow on the panels can hinder sunlight reception, while hail can potentially cause surface damage or micro-cracks, compromising the panel’s reliability.
Suggestion:
For hot climates, choose solar panels with a lower temperature coefficient, such as HJT or IBC panels that perform better under high temperatures. Enhancing ventilation around the panels and using reflective materials or coatings can reduce heat absorption. Against hail or snowy conditions, opt for panels that have undergone rigorous hail resistance testing. Consider installing hail guards or protective nets and invest in insurance for potential hail damage, which can help offset repair or replacement costs.
Conclusion:
When selecting photovoltaic (PV) modules, the operating current, temperature coefficient, spectral response, low-light performance, degradation, installation methods, associated accessories, and external environmental factors of the solar panels are crucial for maximizing the energy output of the solar system. Taking these elements into account holistically can enhance the system’s efficiency and reliability, reduce energy costs, and contribute to the future of clean energy. Through comprehensive planning and selection, we can harness solar resources more effectively and promote sustainable development.
Maysun Solar has been specialising in producing high quality photovoltaic modules since 2008. Choose from our wide variety of full black, black frame, silver, and glass-glass solar panels that utilise half-cut, MBB, IBC, and Shingled technologies. These panels offer superior performance and stylish designs that seamlessly blend in with any building. Maysun Solar successfully established offices, warehouses, and long-term relationships with excellent installers in numerous countries! Please contact us for the latest module quotations or any PV-related inquiries. We are excited to assist you.
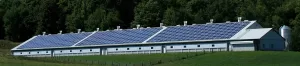
Empowering Factories with Solar Energy A Strategic Tool for Controlling Production Electricity Costs
Commercial and industrial solar is becoming a key solution for factories to reduce electricity costs and hedge against price fluctuations. This article systematically analyzes its deployment models, cost advantages, and sustainable value pathways.
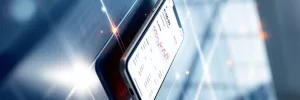
How Businesses Can Offset Carbon Taxes with Solar Power
This article analyzes the latest carbon tax policies and photovoltaic deduction strategies, helping European businesses legally reduce taxes, increase profits through solar investment, and achieve a win-win situation for both economy and environment.
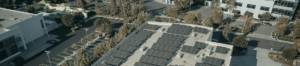
Forecast and Response: Seizing the Next Decade’s Growth Dividend in Europe’s Commercial and Industrial Photovoltaics Market
Maysun Solar analyzes the growth trends of commercial and industrial photovoltaics in Europe over the next ten years, from policies and ESG to technological innovation, helping companies seize the initiative in the energy transition.
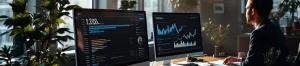
How to Calculate Solar System ROI and Optimize Long-Term Returns?
Solar power is becoming a key solution for businesses to reduce costs and improve efficiency. Accurately calculating ROI and optimizing long-term returns are essential to maximizing investment value.
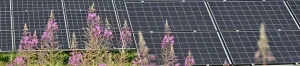
Will Agrivoltaics Affect Crop Growth?
Agrivoltaics combines solar energy and agriculture to reduce up to 700 tons of CO₂ per MW, improve water use, and boost crop growth for sustainable farming.
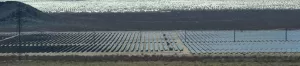
6.5 Billion Loss Hits Photovoltaics: Reshaping or Elimination?
In 2025, the photovoltaic market may see a turnaround as some companies take early action. A €6.5 billion loss is driving businesses to explore new growth areas like energy storage and hydrogen. Which giants will break through? Industry transformation is accelerating!